From summer interns to cubesats in space
By Charlotte E Choudhry, on 7 October 2016
Many students send emails requesting summer internships at UCL Physics & Astronomy, but one particularly caught Dr Anasuya Aruliah’s eye. Vishal Ray was a 2nd year Aerospace Engineering undergraduate at the prestigious Indian Institute of Technology Bombay, India (IIT). He belonged to a student team building their own miniature satellite. Vishal was just the student that Dr Aruliah needed for her new direction of research: satellite drag. After a successful application to the International Students Dean’s Summer Student Scholarships, he was awarded a 2 month internship in summer 2015.
Dr Aruliah’s group, the Atmospheric Physics Laboratory (APL), is a subgroup of the Astrophysics Group. It has a long history of researching the upper atmosphere using a global circulation atmospheric model. They also operate a network of Fabry-Perot Interferometers (FPIs) in Arctic Scandinavia to observe the aurora. The Earth’s atmosphere is like an onion skin, with the troposphere (the domain of weather forecasters), stratosphere and mesosphere as layers on top of each other. The thermosphere is the final layer of the Earth’s atmosphere, and is the altitude region between 90-400km. Low Earth Orbit (LEO) satellites occupy the top of the thermosphere, and rely on upper atmospheric models to predict their orbits.
Recently the APL group found a discrepancy between measurements of thermospheric winds calculated from Doppler shifts of airglow photons, and winds determined from atmospheric drag on the Challenging Minisatellite Payload (CHAMP) satellite. This is an important puzzle to solve because satellite drag measurements are put into atmospheric models to bring them as close to reality as possible. If the ground and satellite measurements do not agree, then which is correct?
The IIT miniature satellite, commonly called a cubesat, is composed of a single cube, only 30 cm in length, width and breadth, and weighing only 10 kg, as much as a few bags of sugar. Their cubesat is called Pratham. This fits perfectly with UCL’s involvement in the European Union FP7-funded QB50 project, in which fifty cubesats carrying miniaturised sensors will be launched nearly simultaneously. This is an international collaboration involving many universities, academic institutes and the space industry. It is an unprecedented science operation, with potential for future Space Weather monitoring campaigns. The QB50 cubesats will be carried by rocket into the upper thermosphere, and fall to Earth in decaying orbits while sampling regions of the thermosphere and ionosphere that were previously poorly understood owing to the lack of detailed measurements.
“The simplicity and low cost of cubesats has spurred much excitement and creativity amongst young (and old) engineers and scientists over the last few years. There are new frontiers being opened by this miniaturised space technology,” said Dr Aruliah.
The UCL Mullard Space Science Laboratory (MSSL) designed and built one of the three key sensors: the Ion Neutral Mass Spectrometer, which will be carried on several of the cubesats, as well as their own cubesat called UCLSat. The QB50 cubesats are scheduled for launch in three batches over the winter period of 2016-2017. Two batches from a Ukrainian-Russian Dnepr rocket, and a third from the International Space Station. During Vishal’s internship at UCL he met with the MSSL cubesat and sensor team, led by Mr Dhiren Kataria and Dr Rob Wicks; and with Dr Stuart Grey in the UCL Department of Civil, Environmental & Geomatic Engineering.
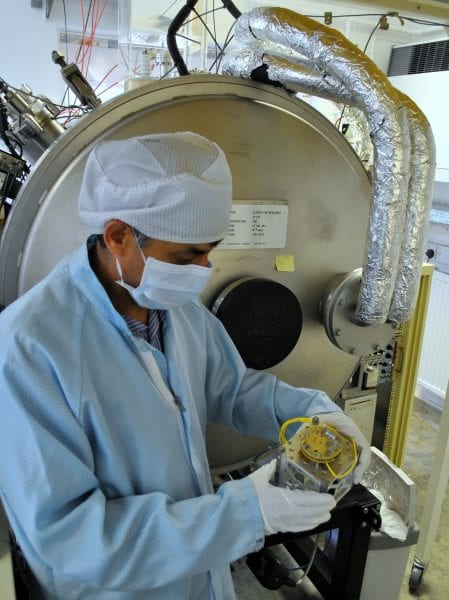
Dhiren Kataria holding the UCLSat designed and built at MSSL
Vishal used his experience to write several sophisticated computer programs to calculate drag coefficients from simulations of a cubesat orbiting in our 3-dimensional atmospheric model called CMAT2. This work was subsequently built upon by Dr Aruliah’s 4th year project student, Jennifer Hall. Jennifer wrote her own programs to derive and compare satellite drag coefficients from CMAT2 simulations and EISCAT radar measurements. Jennifer’s project won the UCL Physics & Astronomy Tessela prize for best use of computer technology in a 4th year project.
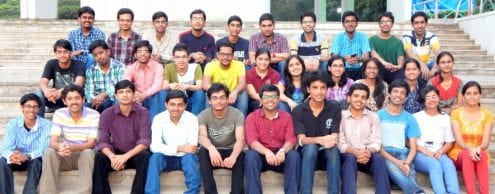
Pratham team at the Indian Institute of Technology Bombay. Vishal Ray is 2nd from the right in the top row.
One year on, after a busy 3rd year of studies, Vishal has written up his summer project as a journal paper, and “Pratham” was scheduled for launch at 0530 UTC on the 26 September 2016. The IIT Bombay student team installed their cubesat on the launch vehicle PSLV C-35 on the remote island of Sriharikota in South India. Vishal said that he “…had goosebumps when we actually placed the satellite on the launch vehicle module and completed the testing for one last time!”. “Pratham” was successfully launched and will measure the total electron count from 800 km altitude in a Sun Sychronous Orbit. MSSL were the first to receive Pratham’s beacon signal, which the students were incredibly excited to hear. You can hear the cubesat from 4:20 onwards as it passes within range of the detector at MSSL. The signal is decipered as “Pratham IIT Bombay Student Satellite”. The accompanying image is of Theo Brochant De Viliers (MSSL) beside the MSSL receiver.
https://soundcloud.com/uclsound/signals-from-the-pratham-cubesat-satelllite
The prospect of finally being launched is very exciting, with both projects having been nearly 10 years in the making. Once launched, the missions will change from the technical challenges of the innovation of miniature sensor devices to the scientific challenges of collecting, analysing and interpreting the measurements. The rewards will be great: from the new technologies surrounding cubesats; to the training of future space scientists and engineers, and to the Space Weather community.