All set for Saturn
By Oli Usher, on 19 June 2015
The Cassini probe launched in October 1997 and arrived in the Saturn system in July 2004. Its Huygens lander touched down on Titan, Saturn’s largest moon, in December 2004. The photo above shows the spacecraft shortly before launch.
More than a decade on, the probe is still sending back data, teaching us about Saturn and its moons.
One of the instruments on board, the Cassini Plasma Spectrometer (CAPS) was designed in part by Prof Andrew Coates at UCL’s Mullard Space Science Laboratory. This week, he has published new research based on data from his instrument.
He has found that the interaction between the Sun, magnetic field lines and the atmosphere of Titan – Saturn’s oddly Earth-like moon – behaves similarly to what we observe here on Earth.
Ferrocene crystals
By Oli Usher, on 10 June 2015
These two images show crystals grown by 2nd year undergraduates in UCL’s chemistry department, as part of their practical classes this week.
The orange grains in the image above are crystals of ferrocene; the darker granules below are made up of the related substance ferrocenium hexafluorophosphate (also known as ferrocenium FP6)
High resolution images
The LHC is back in operation at record energy
By Oli Usher, on 3 June 2015
After two years of repairs and upgrades, the Large Hadron Collider (LHC) is back in operation – and UCL scientists are at the heart of the action. Engineers at CERN confirmed today that the beams of protons that circle in the 27km tunnel near Geneva are stable, and scientific data is once again being collected.

The ATLAS experiment is made of concentric rings of detectors (the particle beam passes through the centre), as seen here during shutdown in 2008. Credit: CERN/Claudia Marcelloni De Oliveria (CERN licence)
UCL scientists have been closely involved in the design, construction and operation of ATLAS, one of the giant detectors that track the high-energy particle collisions in the LHC, so this is an important milestone for the university’s High Energy Physics group.
Until now, the LHC has not been operating at full power. The faults that led to its shutdown shortly after it was inaugurated in 2008 meant that it could only be used to accelerate particles to around half the energy it was designed for.
* * *
Einstein’s equation E=mc2 states that energy and matter are interchangeable.
Atom bombs, famously, create vast amounts of energy by destroying small amounts of matter.
Particle accelerators like the LHC do the opposite, pumping vast amounts of energy into tiny particles, making them move at close to the speed of light. When they collide together, some of that energy is converted into extra matter, in the form of new particles flung out from the site of the collision. The greater that energy, the heavier the particles that can be generated.
Physicists describe the world around us using the ‘standard model’ of particle physics, a set of a handful of particles which can explain the properties and makeup of all the matter and energy we see around us.
The last of those particles to be detected in the lab was the Higgs boson, discovered at CERN in 2012 shortly before the upgrade began.
This doesn’t mean there is nothing left to discover, though. Scientists, including CERN’s director, have begun speaking of a tantalising ‘new physics’ – whole new uncharted areas of science that are currently totally unknown, but which might be explored with higher-energy collisions and heavier particles generated.
One of these areas could be a solution to the riddle of dark matter.

Dark matter can be detected by astronomers (as seen in this Hubble image of a galaxy cluster), but it has not been spotted on Earth, and is known to not be made out of any of the particles in the standard model. Credit: NASA/ESA (CC-BY)
Dark matter has been detected in distant galaxies, thanks to its gravitational effects, but astronomers have determined that it cannot be made of any of the particles described in the standard model.
ATLAS, the building-sized instrument that UCL participates in, played a key role in the Higgs boson discovery, and will play a starring role in the future work of the LHC, and could help explain how dark matter relates to the standard model of particle physics.
* * *
The LHC consists of two 27km-long pipes that use powerful magnets to accelerate beams of protons in opposite directions.
Inside ATLAS, the two beams are brought together on a collision course. The beams are not continuous: the protons come in pulses (“bunches”) about 10cm long and the width of a human hair, each containing around a hundred billion particles. When these bunches cross each other, protons collide and new particles cascade out through the concentric rings of detectors that make up the ATLAS experiment.
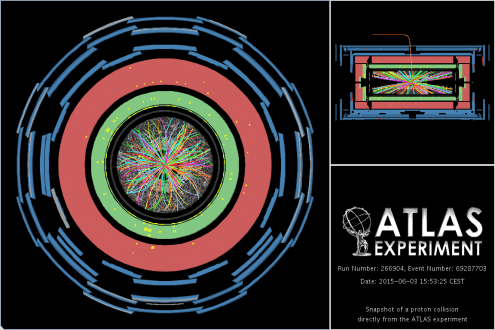
One of the detections made by ATLAS today. This picture is a cross-section of the instrument, with each concentric ring detecting particles’ location or energy, and the particles’ tracks (shown as multi-coloured curved lines) inferred from this data. Credit: CERN (CC BY SA)
Scientists can then trace the path that the particles took – and determine their energy, mass and electrical charges. And from those, they can infer the process that take places in each proton-proton collision.
During the two-year LHC shutdown, the ATLAS scientists also made several improvements to their detector, most notably with the installation of an extra ring of detectors close to the beam pipe, making it more precise than ever before, and part of the team’s work now that the LHC is running again is to ensure that this is all properly calibrated and working as expected.
When it runs at full capacity, ATLAS detects 40 million particle collisions every second, far more than could ever be studied.
Part of the challenge is to discard the unimportant data so that scientists can focus on what’s important. One of the major contributions UCL scientists have made to ATLAS is to the design and operation of the hardware and software algorithms used to discard trivial events in real time and select only the interesting ones – reducing 40 million collisions per second down to a far more manageable 1,000 that are recorded offline.
Another challenge is the simple matter of timing and synchronisation.
With millions of events per second, and everything moving at close to the speed of light, untangling the data from different collisions is challenging. ATLAS is still detecting particles ejected by one collision while another is already taking place.
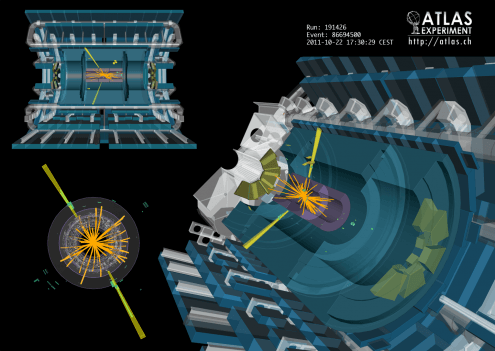
Particles from multiple events cascade through the detectors at one time, and synchronising them is not straightforward. Credit: CERN (CC-BY-SA)
UCL scientists played key roles in developing the electronics that ensure that the data is accurately recorded and readouts from the different components of ATLAS are all kept properly synchronised.
* * *
So what’s next for the LHC?
It’s very hard to say – and that’s what is so exciting about particle physics today.
The standard model is complete. There could be a radical departure, revealing areas of physics never explained before.
Equally, there might just be further confirmation of the dramatic discoveries of the past few decades, giving more precision and certainty to the standard model.
In either case, there is now a chance to explore physics without the constraint of theoretical preconceptions – an unusual and liberating place for a physicist to be.
With thanks to Prof Nikos Konstantinidis for help with this article
How to predict travel chaos
By Oli Usher, on 1 June 2015
It’s a scenario London commuters are all too familiar with: a muffled announcement, ‘signal failure’ or ‘passenger action’; a station closed and thousands of passengers’ journeys interrupted.
London’s transport network is extensive, but fragile.
Everyday disruptions to the service have knock-on effects across the system.
Some are easy to predict – a closure at Mornington Crescent will mean more people exiting at Camden Town, on the same line and only a few minutes’ walk away.

Mornington Crescent: quiet, only on one line, and located near to another station. Photo: Diamond Geezer (CC-BY-NC-ND)
Others are far more complex – how would a closure at a busy hub like Euston affect traffic across the network?
Two innovations of the past few decades mean that travel chaos is far more predictable than it was. One is the wealth of data captured by the Oyster Card system, which has been recording passengers touching in and out of the Underground since 2003. The other is the advance in computing power which makes statistical analysis of millions of journeys easy.
One statistician who has looked at Oyster data to chart the impacts of disruption on the underground is UCL’s Ricardo Silva. He has recently built a statistical model that predicts the knock-on effects of unplanned station and track closures across London’s urban rail network.
His work could help transport operators react more effectively to disruption. It can potentially be used to identify where bus services need to be beefed up, as well as identify bad decisions passengers make when reacting to disruption, which can help station staff make more useful announcements about alternative routes.
At the heart of Silva’s model is a database of every journey taken on the Tube, Overground and DLR using Oyster Cards, over 70 randomly chosen weekdays in 2012 and 2013 – covering tens of millions of passenger journeys.
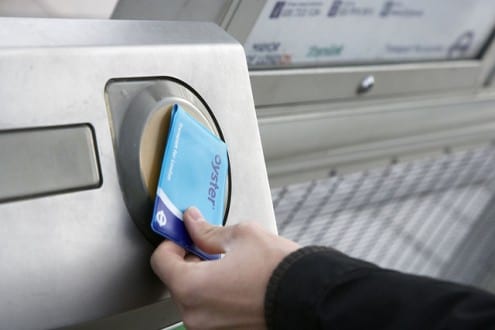
The Oyster Card system is used for over a billion journeys per year on the Tube alone – generating a wealth of data in the process. Photo © TfL Press Office, all rights reserved
Transport for London (TfL) strip out all personal information, such as the passenger’s name or Oyster Card serial number before supplying the data, and give each passenger a randomly allocated ID number. This means that Silva can track individual journeys across the network – including which stations the passengers travel between, and at what time – without invading their privacy.
Alongside the passenger data, he has TfL’s log of all incidents on those days, so he can tease out the difference between passenger behaviour when the network is running smoothly, and when it is being disrupted by a partial closure.
London’s urban rail network has 374 stations, which means there are almost 140,000 possible paths a passenger can take as they navigate their way across the capital. (There are a handful of pairs of stations, Silva says, that nobody travelled between in any of the 70 days he studied.)
Silva’s model predicts minute by minute how many journeys are being made between each pair of stations, how many passengers will enter and leave the system at each station, and how many will be inside the system at any given time.
In its most basic form, the model is simply a description of where passengers are entering and leaving the system at any given time; a reflection of how the network is when it’s working normally. But unlike the real network, he can experiment, closing stations or lines and seeing how the virtual passengers adapt to the disruption.
In principle, the model can give staff immediate feedback about what passengers are likely to be doing at that point, when an unplanned service disruption takes place. However, implementing real time feedback will require further work as the existing technical facilities are not ready for that yet.
Silva’s model takes a lot of computing power – it takes a few days to run a simulation on an ordinary desktop computer – but it is not unmanageable. Extending the model to take account of passenger flows through the transport network, or to run the simulation quicker would require supercomputer facilities such as UCL’s Legion Cluster.
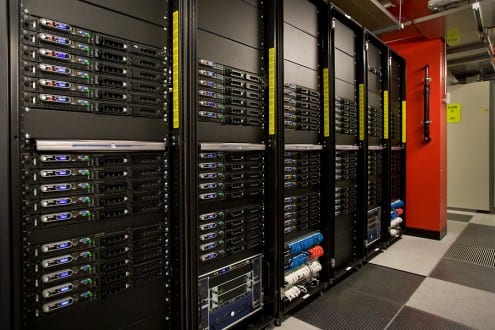
UCL’s Legion Cluster supercomputing facility. Photo: Tony Slade, © UCL Creative Media Services (all rights reserved)
The model doesn’t only apply to London – it is applicable to any transport network where passengers’ entry and exit points are tracked, meaning it could be useful for transport authorities around the world.
Related links
10 Questions
By ucqncca, on 27 May 2015
In this monthly feature, the Institute of Biomedical Engineering (IBME) interviews our researchers, academics, students, clinicians, affiliates and partners to find out a little more about who they are and what they do.
This month we interviewed Professor Allen Goodship, retired as the Director of Institute of Orthopaedics in 2011 and currently part-time Professorial Research Associate in Medical Physics. We asked him 10 questions around his research, career and personal life. Here are his answers….
1) How long have you worked as a Professorial Research Associate?
I first joined UCL as a joint appointment with Royal Veterinary College in 1996. Then was appointed as Director of UCL Institute of Orthopaedics and Musculoskeletal Science in 2000 up to retirement in 2011. I returned in 2012 to the part-time Professorial Research Associate position, enabling me to progress research with minimal administrative duties! Just like being a post doc again!
2) What keywords would you pick to describe your work?
Exciting; interesting; intriguing; challenging, with a touch of frustration.
3) What brought you to the world of science/engineering/medical technologies/medicine?
A long standing fascination of form/function interactions in biological systems in general and the adaptive self-optimisation of skeletal architecture in particular.
4) What is your favourite thing about your work?
The constant challenge in addressing exciting, unanswered questions, and the opportunity to meet a wide range of academics around the world, leading to fantastic research collaborations and lifelong personal friendships.
5) What’s been your career highlight?
Probably the unique experience of participating in a project on the MIR space station, where the science was complemented with the opportunity to undertake some experience of microgravity by flying the “vomit comet”!
Also, having the opportunity to contribute in several areas of research leading to new treatment and diagnostic modalities for diseases in both animals and man.
6) What is your favourite quote?
Probably several of “Peter’s Laws”. In particular, number 5: “Do it by the book …… but be the author!”
7) What’s the best piece of advice you’ve been given?
Look and listen; not always easy to follow!
8) Who has been your greatest mentor and why?
My PhD supervisor – an excellent scientist, fantastic mentor, and lifelong friend. After qualifying as a vet and in my first job in mixed practice, he persuaded me to return to research and academia for which I have no regrets.
On a more personal but “higher” level – my wife Dawn, who has supported me throughout my career.
9) What do you do in your spare time?
Off shore sailing, off road cycling, restoration of vintage motor cycles and more recently, beekeeping – a fascinating hobby, much like academic management, where you think you are in control but actually the bees do exactly as they please! Also, spending time with my grandchildren and family.
10) If you had a superpower, what would it be and why?
Topically, regeneration – to address my increasing interest in aging and to restore normality in disease and degenerative conditions.
Professor Allen Goodship graduated in veterinary science in 1972, his PhD thesis in 1976 related to functional adaptation of bone, both at the University of Bristol. In 2000 he was appointed as Director of the Institute of Orthopaedics and Muscloskeletal Science at UCL and held this position up to retirement in 2011. He returned part time as a Professorial Research Associate at UCL in 2012; in 2015 he transferred to the Department of Medical Physics and Biomedical Engineering.
Soapbox science: bringing science to the people
By ucfbedg, on 22 May 2015
The planet Mercury is approximately 48 million miles away, but this summer I’m bringing Mercury to the SouthBank!
My name is Amy Edgington, I am a PhD student in the Earth Sciences department here at UCL, and I have been lucky enough to be selected as one of the speakers for Soapbox Science in London on 30 May.
Soapbox Science is a series of public outreach events happening all over the country this summer, promoting female scientists and the incredible work they are doing. It’s a great opportunity to share my research with the general public, answer questions, and engage in exciting debate!
Soapbox Science in London, sponsored by L’Oreal, ZSL, STFC and NERC, will transform the SouthBank into an arena for scientific discussion and learning. The variety of topics on show is massive- so there will definitely be something for everyone!
I will be taking to the soapbox to discuss my PhD research so far, investigating the interior of the planet Mercury. The large uncompressed density of the innermost planet suggests it is highly enriched in metallic iron, however, there are ground based measurements1 that imply a liquid layer remains in its interior.
Even just these two clues start to build an intriguing picture of the structure and dynamics hidden deep beneath Mercury’s cratered surface. As part of the Earth Sciences department here at UCL I use ab initio molecular dynamics to study the behaviour and thermodynamics properties of the materials that might form Mercury’s core, namely liquid iron and liquid iron alloys on the atomic scale.
A better knowledge of these materials may lead to a greater understanding of the interior of the solar systems smallest planet, and hopefully unlock insights into its evolution. I will be discussing all of this and more with the help of some iron bolts and a giant polystyrene Mercury on 30 May, from 2-5pm on the Southbank.
Follow @SoapboxScience and @ES_UCL to keep updated with this event, plus visit soapscience.org for more information on all the SoapBox Science events this summer, and to read some of the amazing Women in Science blogs.
Related Links:
- What is Soapbox Science?
- Investigating Mercury – PhD Amy Edgington is talking about her research and life at UCL.
- Amy Edgington, PhD student at Earth Sciences, UCL
- Amy’s Women in Science Blog: High heels, big computers and planetary size cakes
- Soapbox Science Founders & Sponsors: For Women in Science, Fondation L’OREAL, ZSL, Science &Technology Facilities Council, Natural Environment Research Council
The coolest place in London
By Oli Usher, on 18 May 2015
This photo shows a specialised refrigerator, used for cooling objects to within a fraction of absolute zero, located in the Physics building on UCL’s main campus.
When in operation, the refrigerator is entirely enclosed in a sealed and insulated housing, which has been removed here for maintenance.
As with a household fridge, the temperature drops as you go down – with the highest of the four shelves being at a temperature of about 50 Kelvins (-223 Celsius) and the bottom one at just 0.03 K (-273 C).
The refrigerator is used to cool small objects, such as transistors, down to levels where thermal effects (such as the vibration of the atoms in them) are eliminated, allowing quantum effects to be observed.
Samples can be raised or lowered through each shelf via a circular hole in the centre of the refrigerator.
The refrigerator is cooled by a mixture of liquid Helium-3 and Helium-4. The lowest shelf of this refrigerator has a strong claim to being the coldest place in London (alongside a handful of similar facilities at other London universities).
High resolution images
Related links
X-Ray observations probe Sagittarius A*
By Oli Usher, on 14 May 2015
This image shows the centre of the Milky Way galaxy, as seen in X-rays by the Chandra X-Ray Observatory. X-rays are produced by high-temperature, high-energy phenomena.
Within this region lies Sagittarius A*, the Milky Way’s central black hole.
An international team of astronomers, including UCL’s Silvia Zane, has just published new research on a magnetar (a type of super-magnetic neutron star) that is orbiting Sagittarius A*, explaining why it is cooling far slower than theories suggest.
New space photos from UCL’s observatory
By Oli Usher, on 29 April 2015
The University of London Observatory – UCL’s astronomical observatory in Mill Hill, North London – has to deal with England’s murky skies and London’s bright lights, but it can still make some impressive images. Messier 51, seen in the picture above, is actually not one galaxy but two – a large spiral galaxy (Messier 51a) interacting with a smaller dwarf galaxy (NGC 5195). Over the next few hundred million years, they will merge together into one larger galaxy.
Such mergers are quite common. Large spiral galaxies can absorb dwarf galaxies without major disruption to their shapes, though the (rarer) mergers between similarly-sized galaxies tend to destroy all structure, leaving a largely featureless elliptical galaxy. This will be the fate of the Milky Way when it merges with the Andromeda Galaxy in a few billion years time.
The Messier 51 pair are a popular target for amateur astronomers – on a dark night, even relatively basic telescopes can pick out the very faint comma-shape of the galaxy pair, visible near one end of the Plough (Ursa Major).
The picture is one of several newly processed images just published by UCL’s observatory, based on data gathered by astronomy students. The observatory now routinely archives all the digital data gathered with its Celestron telescopes, which are used intensively for undergraduate teaching. This growing archive of data means that multiple observations can be easily combined into a single image, improving contrast and revealing faint details that would otherwise be invisible.
A selection of several dozen of these images from the observatory, with multiple observations processed and combined to form colour composites, is available online to the public. They are free to reuse and reproduce.
What’s next for Hubble?
By Oli Usher, on 24 April 2015
This is the third and final in a series of posts marking the 25th anniversary of the Hubble Space Telescope. Read the first here, and the second here.
Hubble won’t last forever – electrical faults could render any of its instruments inoperable at any time. Though this has happened before, there is no longer a Space Shuttle that can be launched to send astronauts on a repair mission, so any future instrument failures are likely to be permanent.
Moreover, the telescope needs to reliably and steadily lock onto the position of the astronomical objects it is observing. It does this thanks to gyroscopes dotted around the spacecraft – but these will eventually wear out and fail too. Engineers are quietly confident that Hubble can last till at least 2015, but beyond that, the observatory’s future is unclear.
By the end of 2018, the James Webb Space Telescope should join Hubble in orbit – with hardware built at UCL on board. The Webb telescope is not a like-for-like replacement. Webb will have far more powerful capabilities in infrared light, allowing it to peer deep into dust clouds, observe planetary systems being formed, and the see distant redshifted light of the first galaxies.

UCL’s contribution to the James Webb Space Telescope: the NIRSpec (Near Infrared Spectrograph) calibration assembly. This helps maintain accurate scientific observations. Photo credit: UCL MSSL
But it will not have Hubble’s abilities in ultraviolet and visible light. A new breed of telescopes on the ground, such as the European Extremely Large Telescope and the Thirty Meter Telescope will partly replace Hubble’s visible light capabilities (although not with Hubble’s sharpness).
But when Hubble fails, no telescope in operation or in development will replace its ability to observe ultraviolet light, which is blocked by the Earth’s atmosphere.