An Antarctic answer to a cosmic question
By Oli Usher, on 16 March 2015
It’s a setup worthy of Heath Robinson. In a faraway galaxy, a high energy cosmic ray interacts with a photon, producing a series of particle decays eventually releasing a neutrino. The neutrino then crosses billions of light years of space, punching through dust, gas and nebulae without effect. Eventually it reaches Earth, plunges through our atmosphere and finally meets something it cannot pass through: our planet.
When it reaches Earth, scientists hope to detect it. But the complications are only beginning.
High energy neutrino impacts are rare. A cubic kilometre of matter should typically see one roughly once every century. When it does, it triggers a brief and not particularly bright flash of microwave radiation – at the same wavelength and intensity as the flashes from piezoelectric cigarette lighters.
Detect these rare flashes, and you can detect the impact of neutrinos.
Scientists are patient people, and science is no stranger to large and expensive experiments. But building a detector of a cubic kilometre in size, far away from any sources of accidental interference – then waiting potentially a century or more to see a single result – is not reasonable.
Scientists need, somehow, to use the Earth itself as a giant detector, to increase the odds of seeing one.
Searching for somewhere remote enough that the faint signal doesn’t get drowned out, big enough to act as a natural detector, and dry enough not to absorb the microwaves, has taken UCL’s Ryan Nichol to Antarctica three times in the last decade.
There, ANITA (Antarctic Impulsive Transient Antenna), the instrument he works on, circles the South Pole, suspended from a balloon, monitoring a million cubic kilometres of ice for the telltale flash of microwaves that would signal a neutrino detection.
***
Scientists are targeting neutrinos because of what they tell us about cosmic rays.
Cosmic rays are fast-moving particles – usually protons and ions – that bombard the Earth’s upper atmosphere. Some of these move at exceptionally high speeds, up to 99.99999999999999999999% of the speed of light, giving them vast amounts of kinetic energy. A single one of these particles can have the same energy as a cricket ball travelling at 55 miles per hour. But frustratingly, their origins are shrouded in mystery. They must come from some kind of high-energy phenomenon – such as supernovae, black holes or the centres of galaxies – but we can’t figure out what.
Ultra-high energy cosmic rays can travel a long way, but they can’t travel infinitely far. They can interact with photons (light particles), which are everywhere in the Universe, even the vacuum between galaxies. This means that cosmic rays eventually – after about a hundred million light years – come to a halt.
As a result, all the cosmic rays detected in Earth’s upper atmosphere come from a region that includes our galaxy and a few neighbouring ones, but not from the hundreds of billions of galaxies that lie further away. This is by no means a small volume of space, and yet astronomers have come up empty handed when trying to pinpoint the origins of high energy cosmic rays within our cosmic neighbourhood. They need a way of broadening the search.
By looking for high energy neutrinos instead – which are launched like billiard balls by cosmic rays when they interact with photons, but which, unlike them, can cross the void of space without interacting – particle physicists like Nichol hope to extend the search radius from a few tens of millions of light years to several billion.
This should give them a large enough sample to begin to plot where the highest energy cosmic rays are coming from, and see if they can match this up with known objects in the sky that could be causing them.
But first, they need to develop a reliable way of spotting the neutrinos, which is where ANITA comes in.
And for ANITA to do its job, Nichol needs to get to Antarctica. Even in this age of easy international travel, this is not simple. Without any permanent inhabitants, Antarctica has no towns, isn’t visited by scheduled airlines, and even in the brief Antarctic Summer, is still cold and battered by extreme weather.
***
Nichol’s base of operations in Antarctica is McMurdo Station, the largest scientific research station on the continent. It looks like a cross between a mining town and a scruffy university campus – with halls of residence, cafeterias serving mediocre food, and social life centred on a few slightly disreputable bars.
Located on the coast, and served by several runways, McMurdo is one of the gateways to the constellation of smaller research stations dotted around Antarctica. Its summer population tops 1000 – not just researchers, but engineers, cooks, cleaners, drivers – but most of the scientists are just passing through, waiting for connecting flights to more remote stations where they will carry out their research.
For one group of scientists, though McMurdo is perfectly placed: those carrying out research using balloons. High in the sky above the base, a constant, predictable wind blows. Release a balloon into this, and it will gently float in a giant circle around Antarctica, before returning to where it started about two weeks later.
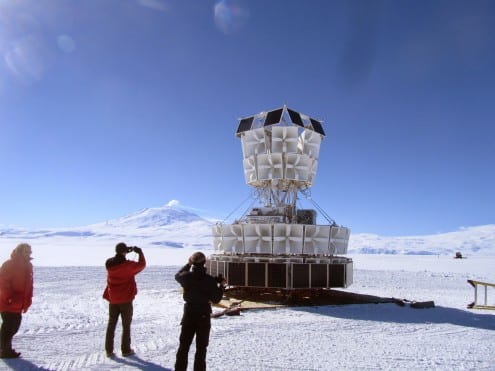
ANITA prior to being attached to the balloon. In the background, Mount Erberus, the second-highest volcano in Antarctica. Photo: Ryan Nichol
For the first couple of days after ANITA is released, McMurdo base is still in range. ANITA floats high in the atmosphere, around three times the altitude of commercial airliners, and so the base, with all the radio interference that comes from it, remains above the horizon for a long time. But once out of range, the only interference is the occasional chirp from communications satellites high above. ANITA silently watches the ice, recording vast amounts of data, and storing it for analysis later on.
The first two flights have not conclusively detected any high energy neutrinos yet. There has been one tentative detection, but the scientists would want to see several before being confident that they hadn’t just seen a false positive.

The flight of ANITA 3 in December 2014/January 2015. The path in red shows the balloon veering off course and ending its journey near Davis base.
This may all change soon. The third flight’s results are on their way home now – and taking a suitably convoluted route back. The December 2014 observations had to be cut short after the balloon blew off course, and the ANITA experiment was jettisoned onto the ice.

ANITA after crash-landing on the ice. Photo: © Josh F/Australian Antarctic Division
Rescued by helicopter and returned to an Australian base, ANITA is now waiting to board the icebreaker Aurora Australis for the journey to Tasmania. The research team hope to get it back next month.
The most recent ANITA mission featured more sensitive instrumentation than the previous two. Given this, the scientists are hopeful – though not certain – that they will get some more tangible results than previous years. They estimate that they should detect somewhere between 0 and 5 high energy neutrino events in this year’s data. So, barring bad luck, the planning, the cold and the years of hard work may just pay off.
***
So if you’re part of the ANITA team, that’s how you detect the highest energy cosmic ray in the Universe. All you need is for it to interact with a photon, emitting a neutrino that flies across billions of years of space before colliding with a water molecule, emitting a flash of microwaves. And for the flash of microwaves to be detected by a sensor suspended from a balloon floating 35km over the most remote and inhospitable region of the world, for the detection to be recorded onto a hard disk, for the hard disk to be dropped onto the ice, then rescued by helicopter, shipped by icebreaker and flown back to the lab, to be decoded by a physicist.
It’s simple, really.
Related links
- ANITA
- Ryan Nichol
- UCL High Energy Physics
- Article on the recovery of the ANITA instrument after its crash landing (including further photos)
Photos